The last year has been extremely busy for me, with (unfortunately) very little time to think about, and draft, new posts for the blog. A lot of interesting things happened, with many changes in the lab and still pending some important news about our research plans and prospects. Besides, it has been a difficult year- especially in summer, with the loss of my mother, a lovely person. So, I very much hope I’ll find more time to keep posting. My apologies for the long silence.
Several interesting items are in the pipeline, including posts about extinct ecological services of Pleistocene megafauna, metanetworks, unveiling long-distance dispersal, multiplex networks and their ecological applications, natural history of junipers, giant lizards, the coevolution of multispecific interactions, and many other themes related to research in the lab. So please stay tuned…
Dodo
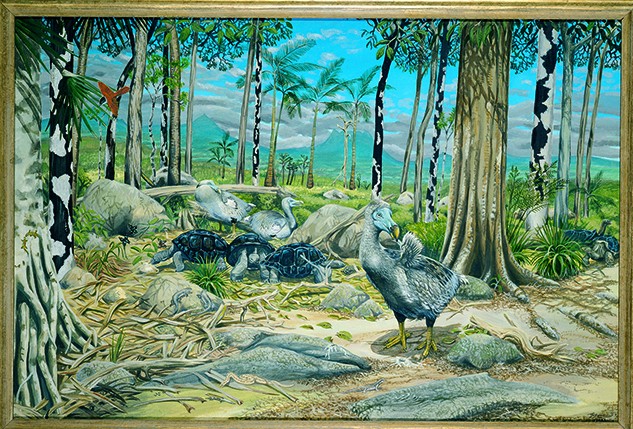
As stated by David Quammen, “the story of the dodo is obscured by a fog of uncertainties.” By about 1690, if not earlier, it was extinct in its area of endemism, Mauritius. Starting around 1500 with the arrival of Europeans, Mauritius, Rodrigues, and Réunion in the Indian Ocean lost 33 species of birds, including the dodo, 30 species of land snails, and 11 reptiles.
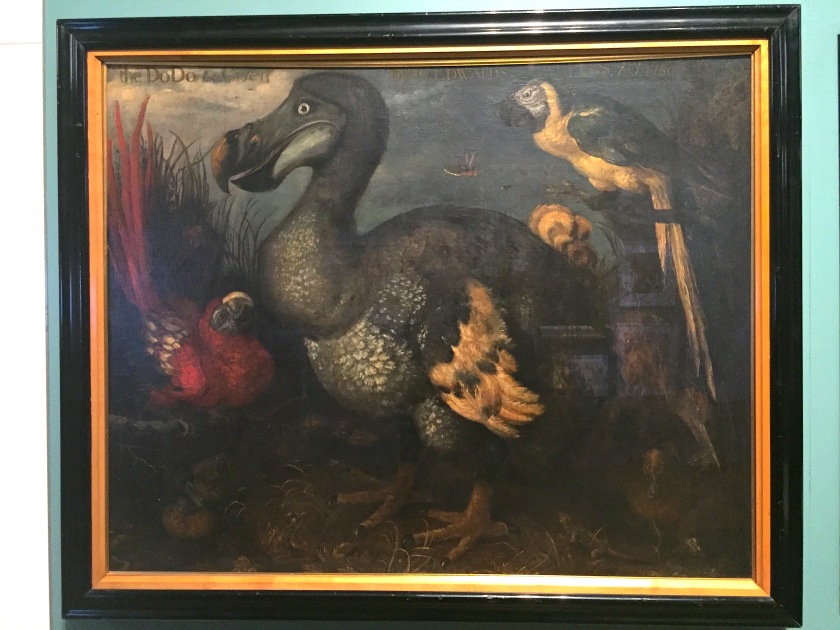
As other large pigeons, especially on islands, dodos probably relied extensively on fruit food. A famous iconic story relates the extinction of dodos, to the almost co-extinction of a seemingly preferred fruiting tree, Sideroxylon grandifolium (formerly Calvaria major, Sapotaceae, the tambalacoque tree), thought to have relied exclusively on these birds for seed dispersal. The tree is an endemic species. According to historical records, it had once been common in upland Mauritian forests and was often exploited for lumber. According to the original hypothesis of coextinction, set by Temple (1977) based on a traditional belief of Mauritius people: “In response to intense exploitation of its fruits by dodos, S. grandifolium evolved an extremely thick endocarp as a protection for its seeds; seeds surrounded by thin-walled pits would have been destroyed in the dodo’s gizzard. These specialized, thick-walled pits could withstand ingestion by dodos, but the seeds within were unable to germinate without first being abraded and scarified in the gizzard of a dodo.”
Dodos were large-bodied birds, averaging- according to the most recent estimates- 10 kg and reaching up to 12 kg (previous estimates reported up to ~21 kg). The beak was very robust, ~5 cm gape width, probably apt to handle and swallow very large fruits as well as other plant material.
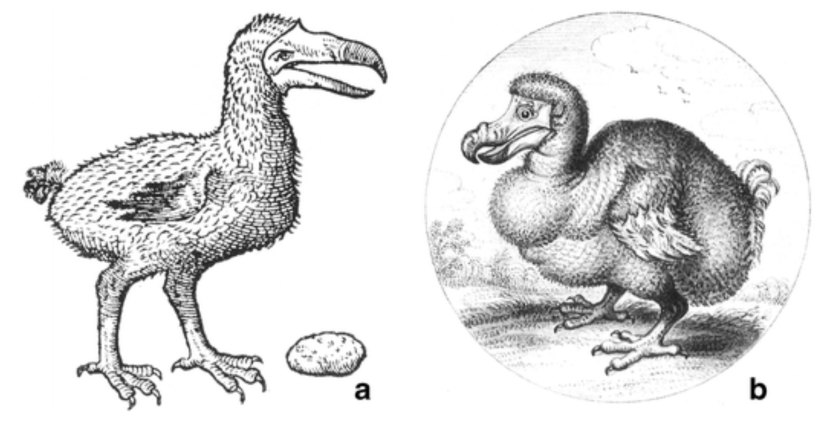
Coextinctions are extremely difficult to demonstrate, especially for interactions involving, e.g., small species (e.g., ectoparasites and hosts) and species involved in generalized interactions (plant-animal mutualisms for pollination and seed dispersal). Yet we have many evidences for functional coextinctions, happening when species become very rare (even extinct) and their ecological roles are lost. Dodos and tambalacoques probably illustrate this. S. grandifolium has persisted on the island likely because of haphazard dispersal by other dispersal agents (e.g., giant skinks and turtles) and rare instances of runoff, etc. Seeds have been found germinating in some cases, yet with very low proportions; while pulp removal was required for germination, it appears that seed scarification does not improve germination significantly. And dodos may had the ability to crack the hard seeds during digestion. Yet there is no proper test available about of all these aspects, as far as I know.

The tree still remains in relict stands but juveniles can be found. The dispersal has certainly collapsed, yet with no final effect entailing the extinction of the tree on the island. Probably many local stands of S. grandifolium have disappeared since dodo’s extinction ca. 400 yr ago. Recent analyses of rich fossil plant and animal remains in lowland Mauritius include many S. grandifolium seeds associated with dodo and giant turtle remains. But it seems we are not in front of a tightly coevolved one-to-one instance of pairwise coevolution. Most likely, not simply the dodo extinction contributed to the rarity of tamabalacoques on Mauritius (and several other large-seeded trees): competition with exotic species, were most likely fundamental. Introduced species included Javan deer, goat, pig, crab-eating macaque, and black rat were clear contributors for the dodo’s extinction by destroying the understory vegetation, competing for food sources, and, in the case of the pig, macaque, and black rat, direct predation on eggs and chicks.
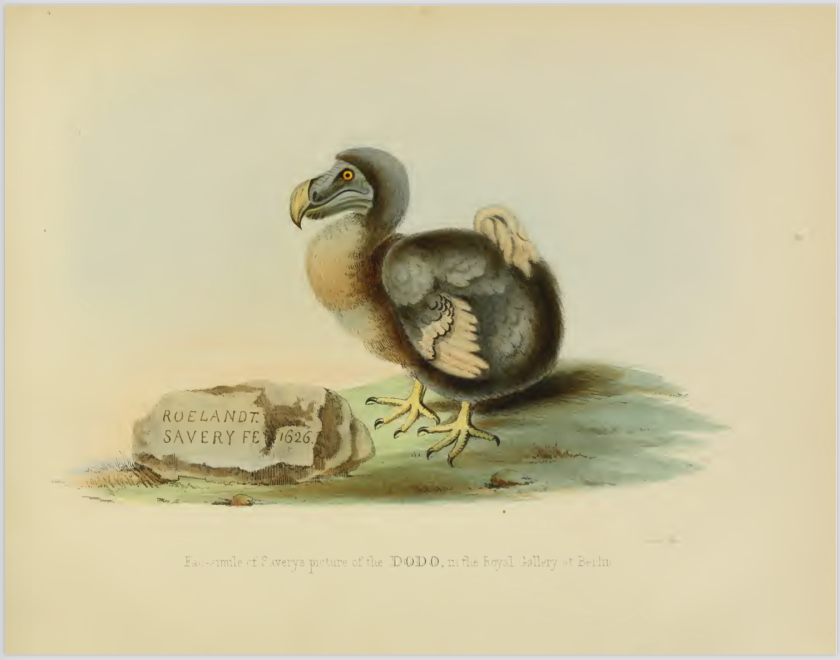
Independently of whether the initial reports of coextinction due to loss of the mutualistic dodo were wrong, or biased, or both, the system reflects what happens when ecological interactions are lost: we simply see highly altered systems that remain extremely difficult- or even impossible- to resurrect. And oftentimes the extinction of interactions precedes by a long time the extinction of species, so that what we see is the pervasive effect of the debt of lost interactions.
- Angst, D., Buffetaut, E. & Abourachid, A. (2011) The end of the fat dodo? A new mass estimate for Raphus cucullatus. Naturwissenschaften, 98, 233–236.
- Herhey, D. (2006) The widespread misconception that the tambalacoque or Calvaria tree absolutely required the dodo bird for its seeds to germinate. Plant Science Bulletin, 50, 105–109.
- Oudemans, A.C. (1917). Dodo-Studien. Johannes Muller, Amsterdam.
- Pimm, S.L. (2002) The dodo went extinct (and other ecological myths). Annals of the Missouri Botanical Garden, 190–198.
- Quammen, D. (1996) The Song of the Dodo. Scribner, NY, USA.
- Rijsdijk, K.F., Hume, J.P., Louw, P.G.B.D., Meijer, H.J.M., Janoo, A., De Boer, et al. (2016) A review of the dodo and its ecosystem: insights from a vertebrate concentration Lagerstätte in Mauritius. Journal of Vertebrate Paleontology, 35, 3–20.
- Strickland, H.E., Melville, A.G. (1848) Dodo and its kindred. History, affinities, and osteology of the dodo, solitaire, and other extinct birds of the islands Mauritius, Rodriguez, and Bourbon. Reeve, Benham and Reeve, London, UK.
- Temple, S.A. (1977) Plant-animal mutualism: coevolution with dodo leads to near extinction of plant. Science, 197, 885–886.
- Witmer, M.C. & Cheke, A.S. (1991) The dodo and the tambalacoque tree: an obligate mutualism reconsidered. Oikos, 61, 133–137.
Text: Pedro Jordano. Illustrations and photos, from digitized original books at Biodiversity Heritage Library, and Spanish National Library. Also, photos by M. Galetti and P. Jordano.
Ginkgo
Ginkgoes and dodos are my two favorite icons of “ghost” mutualistic interactions. So, I had the two last posts on megafauna and extinct interactions dedicated to them.
There are only five living groups of seed plants, and ginkgo is one of them; just a single species. Ginkgoes (Ginkgo biloba, Ginkgoaceae) have fleshy “fruits” and their “seeds” were dispersed by animals including, most probably, from dinosaurs to Pleistocene megafauna, and to extant frugivores nowadays. The reason is that the ginkgo has survived on Earth for a really extended period of time, with the earliest fossils of ginkgo-like plants dated more than 200 million years ago. Among the many ginkgo-like tree species, only Ginkgo biloba has survived (up to five Ginkgo species are known as fossils).
Living ginkgo very nearly went extinct, in fact, as of two million years ago, existing in only a small area in eastern China, the Tian Mu Shan mountains in Guizhou Province. Ginkgoes have then survived just by human intervention, with an assisted dissemination for cultivation starting at least 1200 yr ago by Buddhist monks, and introduced to Europe just by 1730-1750.

Most likely a combination of extreme dispersal limitation due to lack of efficient seed dispersers combined with large-scale climate shifts and habitat modification contributed to their nearly extinction in the wild. Contrary to other tree species, retractions to small refugia populations failed to recover the original range, especially in North America and Europe. As with other megafauna-dependent species, it resprouts vigorously from buds buried in its underground parts, and human use certainly rescued the ginkgoes, probably because of their nutritous “nut”. In Peter Crane’s words: “It is irrepressible; its capacity for self-preservation has helped it survive through millions of generations.”
We know very little about how seed dispersal works in living ginkgo. The fleshy “fruit” is really the mature, fertilized ovule with a a three-layered integument: a fleshy outer sarcotesta, a stony inner sclerotesta, and a thin endotesta. Its smelly, large seeds (20-30 mm x 16-24 mm) are one of its most well-known and distinctive features: the seed’s soft outer layer starts to break down after a few days on the ground and produces butyric acid, CH3(CH2)2COOH giving it the “interesting” odor. Germination improves after the fleshy seed coat has been removed by passing through the gut of an animal or being teared-off. In one of the potentially wild ginkgo populations in China it is documented that the seeds are eaten by a wild cat, and in Japan they are eaten by badgers. Yet, there were very few seedlings in this population, located in 1989 by Del Tredici, despite good fruiting. People harvested the nuts, which are very nutritious, as well as Pallas’s squirrels (Callosciurus erythraeus), which also may act as good dispersers by scatter-hoarding the seeds.
Yet who were the seed dispersers that mediated the range expansion of ginkgoes over continents and islands (Japan) before human-mediated propagation? As in other megafauna-dependent plants, most likely a combination of dispersal agents, including large and medium-sized mammals and, well before that, dinosaurs. As with other extant large-‘seeded’ Coniferopsida like Cephalotaxus and Torreya with very large seeds, scatter-hoarding animals like the extinct multituberculates (i.e., the ‘rodents’ of the Mesozoic; g. Ptilodus) would have played a role in active seed dispersal of ginkgoes by scatter-hoarding the seeds.
We can see ginkgoes as survivors with a long history of mutualistic interactions involving a diverse array of animals, whose actual diversity we can only speculate about, then replaced by extensive human use.
- van Beek, T.A. (2003) Ginkgo biloba. CRC Press, NY.
- Crane, P. (2013). Ginkgo. The tree that time forgot. Yale University Press, New Haven.
- del Tredici, P. (1989) Ginkgos and multituberculates: evolutionary interactions in the Tertiary. Bio Systems, 22, 327–339.
Text: Pedro Jordano with excerpts from Del Tredici (1989) and Crane (2013). Illustrations: WikiMedia.
Megafauna in Madagascar
A scene in Madagascar in the late Pleistocene. From left to right: elephant bird (Aepyornis maximus), Malagasy giant rat (Hypogeomys antimena), melanistic giant fossa (Cryptoprocta spelea), monkey lemur (Archaeolemur), streaked tenrec (Hemicentetes), and koala lemur (Megaladapis).
Madagascar had a highly diversified megafauna, as also occurred in other islands, quickly becoming defaunated because of human action and habitat destruction, starting very recently, ca. 2000 yr BP. For example, the Spiny Thicket Ecoregion (STE) of SW Madagascar was home to numerous giant lemurs and other megafauna, including pygmy hippopotamuses, giant tortoises, elephant birds, and large euplerid carnivores.
Island frugivore faunas are much more phylogenetically diverse than continental ones; their frugivore assemblages are known as disharmonic because a given plant species may depend on distinct sorts of animal seed dispersers (e.g., lizards, birds, mammals) quite distinct in evolutionary history and likely not being complementary in their ecological functions. For example, only one-third of the lemur species which earlier occupied the spiny thicket ecoregion survive today. The extinct lemurs occupied a wide range of niches, often distinct from those filled by non-primates. Many of the now-extinct lemurs regularly exploited habitats that were drier than the gallery forests in which the remaining lemurs of this ecoregion are most often protected and studied. Recent evidence using stable isotope biogeochemistry has shown that most extinct lemurs fed predominantly on C3 plants and some were likely the main dispersers of the large seeds of native C3 trees; others included CAM and/or C4 plants in their diets.
While the negative effects on seed dispersal of Pleistocene megafauna extinction in continental areas were probably buffered by complementary dispersers (e.g., scatter-hoarders, domestic megafauna, human use), island assemblages had not this option. Thus, if we seek instances of actual co-extinction of co-dependent frugivores and their food plants we may probably have to resort to islands, especially oceanic islands, or extreme habitats (e.g., deserts) where the mutualistic partners are highly disharmonic.
- Crowley, B.E., Godfrey, L.R. & Irwin, M.T. (2011) A glance to the past: subfossils, stable isotopes, seed dispersal, and lemur species loss in Southern Madagascar. American Journal of Primatology, 73, 25–37.
- Grubb, P.J. (2003) Interpreting some outstanding features of the flora and vegetation of Madagascar. Perspectives in Plant Ecology Evolution and Systematics, 6, 125–146.
- Jungers, W.L., Demes, B. & Godfrey, L.R. (2007) How big were the “‘giant’” extinct lemurs of madagascar? J. G. Fleagle, C. C. Gilbert (eds.), Elwyn Simons: A Search for Origins. Springer, pp: 1–18.
- Shapcott, A., Rakotoarinivo, M., Smith, R.J., Lysakova, G., Fay, M.F. & Dransfield, J. (2007) Can we bring Madagascar’s critically endangered palms back from the brink? Genetics, ecology and conservation of the critically endangered palm Beccariophoenix madagascariensis. Botanical Journal of the Linnean Society, 154, 589–608.
Text: Pedro Jordano; excerpts fromCrowley et al. 2011.
Illustration: William Snyder @deviantart.com (Pleistocene Madagascar).
It takes guts to disperse seeds: the amazing physiologies of megafauna
Megafauna can be divided in two large groups in terms of food digestion: foregut and hindgut fermenters, depending on where in the digestive tract the ingesta is digested. Foregut fermenters include ruminants, pseudoruminants (i.e., hippo, camelids), just the hoatzin among birds, and the colobine monkeys, sloths, and some marsupials and rodents- all them have complex, multipart stomachs. Hindgut fermenters are monogastric herbivores.
The very large megafauna are largely non-ruminants and may have either foregut or hindgut fermentation of food, with this having very important consequences for seed treatment. While the largest extant non-ruminant foregut fermenter is the hippopothamus, the largest terrestrial animals nowadays are hindgut fermenters, with the exception of large bovids: elephants, rhinos, equids, tapirs.
Interestingly, the digestive tract of elephants is surprisingly short compared to other herbivorous mammals. Typical retention times of ingesta in elephants are below 50h; with Asian elephants achieving higher digestion coefficients on comparable diets, and having longer ingesta mean retention times, than their African counterparts. This is probably associated to the fact that intestine lengths of Asian elephants (~30m) nearly double those of African elephants (~15m) for a given body mass.
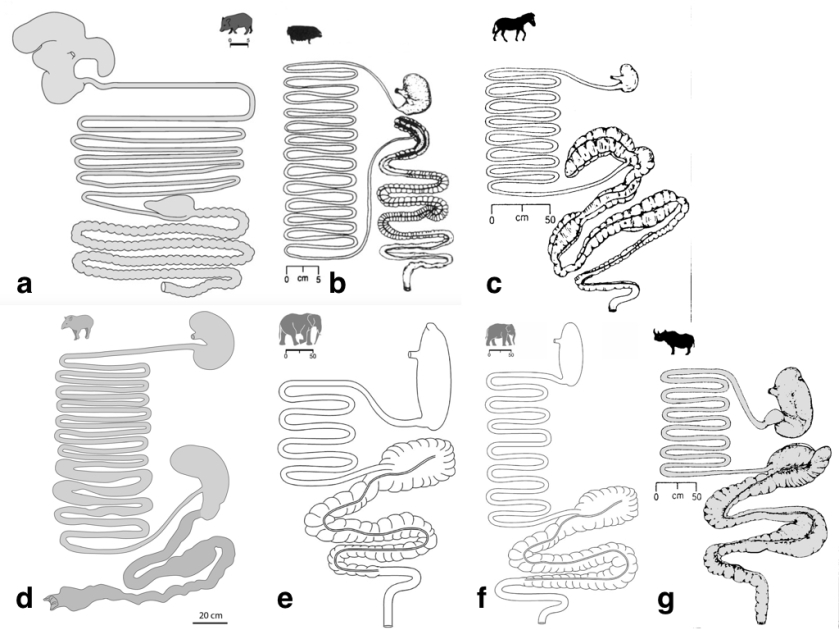
Tapirs, in the order Perissodactyla, are the closest extant relatives to equids and rhinoceroses, thus their digestive tract reportedly resembles that of horses. They both have a large caecum and proximal colon as fermentation chambers. In both the horse and the rhinoceros, the caecum and colon have approximately the same width. In contrast, the tapir also has a large caecum, but the rest of the large intestine—in particular, the ventral proximal colon— is less voluminous. The caecum of the tapir is its most voluminous gastro-intestinal section, suggesting that during the evolutionary history of tapirs, and in contrast to other extant perissodactyls, the caecum was the major fermentation site in the digestive tract.
The caecum of rhinos, horses, and probably also tapirs may retain seeds for many days (kind of a side-storage of indigestible food), being suddenly evacuated in pulses. The browsing black rhinoceros (Diceros bicornis) has both shorter small and large intestines than the grazing rhinoceroses (Ceratotherium simum, Rhinoceros unicornis).
Peccaries in contrast, are foregut fermenters, with a digestive tract characterised by an elaborate forestomach. Peccaries have a small relative stomach volume compared to other foregut fermenters, which implies a comparatively lower fermentative capacity and thus forage digestibility. The forestomach could enable peccaries to deal, in conjunction with their large parotis glands, with certain plant toxins (e.g. oxalic acid).
This fascinating diversity of digestive strategies and food processing has undoubtely emerged from coevolved interactions with plants, either as antagonistic herbivores or mutualistic seed dispersers. Plants were benefited by megafauna evolving very large body sizes (especially among monogastric hindgut fermenters), yet with relatively short retention times that did not damage seeds, even with a lengthy digestion process; however, with more limitations to detoxify plant toxins compared to ruminants. Many of the extremely large extinct megafauna (e.g., Indricotherium, reaching up to 15000 kg body mass) were most likely hindgut fermenters with browsing habits and extensive use of fruit food. Ruminants, on the other hand, have been likely limited in their evolution to smaller body sizes (up to 1200 kg in some bovids, 2700 kg in hippos). All the very large ruminants (bovids, buffalo, zebu), but not the smaller ones (e.g., antelopes) lack the ability to reabsorb water in the colon and depend on the availability of drinking water.
The combinations of digestive characteristics of monogastric hindgut fermenters supports their key ecologial functions for seed dispersal: 1) ample diversity of plant food species dispersed; 2) extremely large number of seeds dispersed due to huge gut capacities; 3) long seed dispersal distances due to long retention times with a distinct role of caeca; and 4) gentle treatment to seeds during mastication and digestion, favouring adequate germination potential of dispersed seeds in most instances.
Photos: Kulpat Saralamba, Kim McKonkey, Mauro Galetti, Carlos R Brocardo, WikiCommons.
- Clauss, M. & Hummel, J. (2005) The digestive performance of mammalian herbivores: why big may not be that much better. Mammal Review, 35, 174–187.
- Clauss, M., Steinmetz, H., Eulenberger, U., Ossent, P., Zingg, R., Hummel, J. & Hatt, J.M. (2006) Observations on the length of the intestinal tract of African Loxodonta africana (Blumenbach 1797) and Asian elephants Elephas maximus (Linné 1735). European Journal of Wildlife Research, 53, 68–72.
- Clauss M, Steuer P, Müller DWH, Codron D, Hummel J (2013) Herbivory and body size: allometries of diet quality and gastrointestinal physiology, and implications for herbivore ecology and dinosaur gigantism. PLoS One 8:e68714
- Hagen, K., Müller, D.W.H., Wibbelt, G., Ochs, A., Hatt, J.-M. & Clauss, M. (2014) The macroscopic intestinal anatomy of a lowland tapir (Tapirus terrestris). European Journal of Wildlife Research, 61, 171–176.
- Müller, D.W.H., Codron, D., Meloro, C., Munn, A., Schwarm, A., HUMMEL, J. & Clauss, M. (2013) Assessing the Jarman–Bell Principle: Scaling of intake, digestibility, retention time and gut fill with body mass in mammalian herbivores. Comparative Biochemistry and Physiology, Part A, 164, 129–140.
- Schwarm, A., Ortmann, S., Rietschel, W., Kühne, R., Wibbelt, G. & Clauss, M. (2009) Function, size and form of the gastrointestinal tract of the collared Pecari tajacu (Linnaeus 1758) and white-lipped peccary Tayassu pecari (Link 1795). European Journal of Wildlife Research, 56, 569–576.
Moas
The diversity of moas was extraordinary, including nine (10, for some authors) species in six different genera, all them endemic to New Zealand. They nicely illustrate megafaunal birds, whose extant furgivorous relatives include cassowaries and, with partial frugivory, kiwis.
Moas weighted 35-250 kg, becoming extinct ~1300-1440 yr BP due to overhunting. Moas included at least three distinct assemblages in the South Island during the Holocene: a wet western beech forest assemblage consisting of South Island giant (Dinornis robustus) and little bush (Anomalopteryx didiformis) moas; an assemblage from the relatively dry eastern forests and scrublands, consisting of South Island giant, eastern (Emeus crassus), heavy-footed (Pachyornis elephantopus) and stout-legged (Euryapteryx gravis) moas; and an assemblage from upland and subalpine areas consisting of South Island giant, crested (Pachyornis australis) and upland (Megalapteryx didinus) moas.
The discovery in New Zealand of Late Holocene deposits of coprolites from these extinct avian megaherbivores has provided a unique opportunity to gain a detailed insight into the ecology of these birds across ecologically diverse habitats. Macrofossil analysis of 116 coprolites of the giant ratite moa (Aves, Dinornithiformes) reveals a diverse diet of herbs and low shrubs in both semi-arid and high rainfall ecological zones, overturning previous models of moa as dominantly browsers of trees and shrubs.
Ancient DNA analysis identified coprolites from four moa species (South Island giant moa, Dinornis robustus; upland moa, Megalapteryx didinus; heavy-footed moa, Pachyornis elephantopus and stout-legged moa, Euryapteryx gravis), revealing a larger dietary variation between habitat types than between species. Most species of moa, if not all, included fruit in their diets, and may have been also important as dispersers of grass seeds.
The new data confirm that moa fed on a variety of endemic plant taxa with unusual growth forms previously suggested to have co-evolved with moa. Lastly, the feeding ecologies of moa are shown to be widely different to introduced mammalian herbivores. The broad range of feeding strategies used by moa, as inferred from interspecific differences in biomechanical performance of the skull, provides insight into mechanisms that facilitated high diversities of these avian megaherbivores in prehistoric New Zealand.
- Atkinson, I.A.E. & Greenwood, R.M. (1989) Relationships between moas and plants. New Zealand Journal of Ecology, 12, 67–96.
- Attard, M.R.G., Wilson, L.A.B., Worthy, T.H., Scofield, P., Johnston, P., Parr, W.C.H. & Wroe, S. (2016) Moa diet fits the bill: virtual reconstruction incorporating mummified remains and prediction of biomechanical performance in avian giants. Proceedings of the Royal Society of London Series B-Biological Sciences, 283, 20152043–9.
- Bond, W., Lee, W. & Craine, J. (2004) Plant structural defences against browsing birds: a legacy of New Zealand’s extinct moas. Oikos, 104, 500–508.
- Burrows, C.J., McCulloch, B. & Trotter, M.M. (1981) The diet of moas based on gizzard contents samples from Pyramid Valley, North Canterbury, and Scaife’s Lagoon, Lake Wanaka, Otago. Records of the Canterbury Museum, 9, 309–336.
- Lee, W.G., Wood, J.R. & Rogers, G.M. (2010) Legacy of avian-dominated plant-herbivore systems in New Zealand. New Zealand Journal of Ecology, 34, 1–20.
- Wood, J.R., Rawlence, N.J., Rogers, G.M., Austin, J.J., Worthy, T.H. & Cooper, A. (2008) Coprolite deposits reveal the diet and ecology of the extinct New Zealand megaherbivore moa (Aves, Dinornithiformes). Quaternary Science Reviews, 27, 2593–2602.
- Wood, J.R., Wilmshurst, J.M., Richardson, S.J., Rawlence, N.J., Wagstaff, S.J., Worthy, T.H. & Cooper, A. (2013) Resolving lost herbivore community structure using coprolites of four sympatric moa species (Aves: Dinornithiformes). Proceedings of the National Academy of Sciences USA, 110, 16910–16915.
The Gardeners of the Forest
Elephants are the major gardeners of the rainforest. Weighing around 4000 kg, they are more than twice as large as the next biggest sympatric animal species (the one-horned rhinoceros) and four times as large as the third-place finisher (the gaur, Bos gaurus). Current taxonomy recognizes two extant species of elephant, the African elephant (Loxodonta africana), with forest and savannah subspecies, and the Asian elephant (Elephas maximus). They disperse massive amounts of seeds in conditions adequate for germination and establishment of tree seedlings, with estimates ranging between 300-2000 seeds/km2/day depending on elephant species and habitat. Recent studies indicate that seeds taken from elephant dung germinated as well or better than seeds from bovid dung or directly from fruit. Elephants were calculated to move seeds up to 10 times as far as domestic bovids. When elephants are missing, there are no ecological counterparts to compensate their absence.
The video from the Elephant Reintroduction Foundation nicely cartoons the type of mechanistic models that help us to estimate the ecological functions derived from mutualistic interactions between these megafrugivores and plants.
An empirical probability model estimated that the loss of elephants would result in reductions of about 66%, 42%, and 26% in the number of successfully dispersed seeds of key species such as Dillenia indica (chalta), Careya arborea (kumbhi), and Artocarpus chaplasha (lator), without compensation. In compensation scenarios, other frugivores could ameliorate reductions in dispersal, making them as low as 6% if species such as gaur (Bos gaurus) persist. Thus the importance of elephants as seed dispersers is amplified by the population reductions of other large disperser species throughout tropical Asia. The African and Asian elephants are the exclusive or near-exclusive disperser of a considerable number of plant species. The loss of forest elephants (and other large-bodied dispersers) may lead to a wave of recruitment failure among animal-dispersed tree species, and favor regeneration of the species-poor abiotically dispersed guild of trees.
– Beaune, D., Fruth, B., Bollache, L., Hohmann, G. & Bretagnolle, F. (2013). Doom of the elephant-dependent trees in a Congo tropical forest. Forest Ecology and Management, 295, 109–117.
– Blake, S., Deem, S.L., Mossimbo, E., Maisels, F. & Walsh, P. (2009) Forest elephants: tree planters of the Congo. Biotropica, 41, 459–468.
– Campos-Arceiz, A., & Blake, S. (2011). Megagardeners of the forest – the role of elephants in seed dispersal. Acta Oecologica, 37, 542-553.
– Sekar, N., Lee, C.L. & Sukumar, R. (2015). In the elephant’s seed shadow: the prospects of domestic bovids as replacement dispersers of three tropical Asian trees. Ecology, 96, 2093–2105.
– Sukumar, R. (2003). The living elephants: evolutionary ecology, behavior, and conservation. New York: Oxford Univ. Press.
The Cryptic Extinctions
McConkey, K.R. & O’Farrill, G. (2016) Loss of seed dispersal before the loss of seed dispersers. Biological Conservation, 201, 38–49.
Cryptic function loss is a loss in the function of a species that is hidden by its continued presence in the ecosystem; the species may still be present and showing up in a biodiversity inventory, yet its functional ecological role has disappeared.
The authors reviewed the evidence for cryptic function loss to be widespread among seed disperser populations that persist under disturbed conditions. The results overwhelming support for the seed dispersal effectiveness of animals to be negatively impacted by all forms of disturbance (population decline, changes in community assemblages, habitat change, and climate change). However, seed dispersal was positively affected in some examples, particularly when extirpation of an interacting frugivore or predator enhanced fruit consumption.
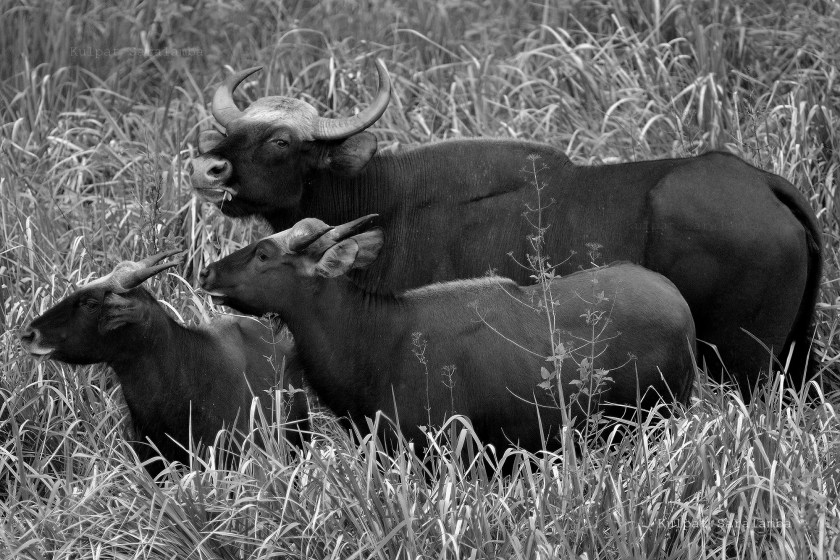
Behavioral changes are usually the first adaptation of an animal to disturbance and are likely to be a common trigger for function loss without species loss, resulting in the animal no longer performing a function carried out previously. Substantial decrease in population density or demography of an animal species can also trigger cryptic function loss; for example, when specialization occurs among individuals within a single population and a non-random subset of individuals is particularly vulnerable to a disturbance. Finally, cryptic function loss can occur through phenotypic adaptation of an animal species, when the physical alteration enhances survival but is not matched by interacting species.
Given the ample generalization shown by many seed dispersal systems, we are far from understanding the consequences of functional losses due to population density collapses of frugivore species triggered by disturbances. In Dan Janzen’s words, this is the most pervasive kind of extinction, the extinction of interactions.
See also:
– Jarić, I., 2015. Complexity and insidiousness of cryptic function loss mechanisms. Trends in Ecology and Evolution 30, 371–372.
– Valiente-Banuet, A., Aizen, M.A., Alcántara, J.M., Arroyo, J., Cocucci, A., Galetti, M., García, M.B., García, D., Gomez, J.M., Jordano, P., Medel, R., Navarro, L., Obeso, J.R., Oviedo, R., Ramírez, N., Rey, P.J., Traveset, A., Verdú, M., Zamora, R., 2015. Beyond species loss: the extinction of ecological interactions in a changing world. Functional Ecology 29, 299–307.
Text: Excerpts from McConkey, K.R. & O’Farrill, G. 2016; and Pedro Jordano. Photo: Kulpat Saralamba.
Extant Megafauna Frugivores
The end-Pleistocene mega-mammal extinction (also including other vertebrate groups) likely had a severe effect on present-day megafauna assemblages and impaired important functions associated with ecological interactions involving megafauna taxa. The extant mega-mammal faunas around the world are impoverished versions of the Pleistocene biota on most continents except- perhaps- Africa. In addition, mega-mammals are particularly hard hit by ongoing human-driven disturbances like deforestation, hunting, pollution, and animal trade.
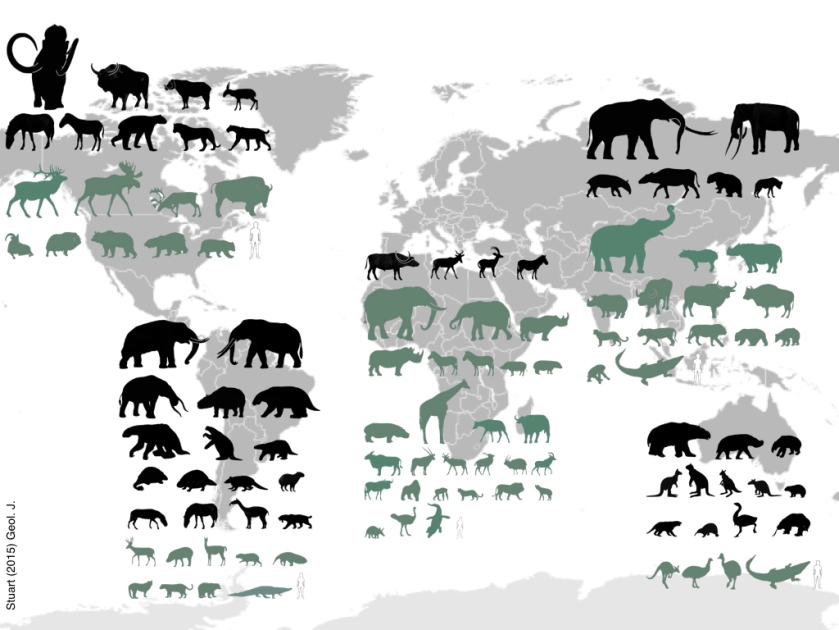
Extant frugivorous mega-mammals are represented in a few orders and families: Carnivora, Artiodactyla, Perissodactyla, Marsupalia, Proboscidea, and Primates. Yet they span a high diversity of body sizes, digestive systems, movement patterns, and foraging modes, presumably defining a wide range of ecological functions for plant dispersal.
The representation of extant mega-birds is much more restricted- strictly speaking, to the large Ratites (emus, cassowaries, ostrich) most of them consuming fruits to variable extents.
Then, herps and fish have also a reduced representation, with large iguanas, varanid lizards and giant turtles, on one hand, and a few genera of very large frugivorous fishes.
The population densities, distribution areas, and even body sizes of these extant megafauna species are being severely reduced by both direct and indirect human influences. This is what we call the anthropocene, and the defaunation events associated to global change drivers such as deforestation. We are just starting to grasp the delayed consequences of this dramatic loss of biodiversity for the persistence of forests worldwide.
Illustration: Pedro Jordano, based on Stuart (2014). Photos: Kulpat Saralamba, Alicia Solana, Néstor Pérez-Méndez, Dennis Hansen.
Megafauna-Dependent Plants
How did megafauna-dependent plants survive after the demise of the giant Pleistocene seed dispersers? Before hand, be warned that coextinctions are very difficult to assess and demonstrate in nature, especially for certain groups (e.g., hosts and ectoparasites). Moreover, think of the myriad possibilities for plants to stay on place even with collapsed dispersal: just haphazard seed dispersal may help; or suboptimal fruit removal and sporadic dispersal by other, less reliable frugivores; or the dispersal being taken over by efficient frugivores (e.g., scatter-hoarders) yet with limitations in some aspect of the dispersal service (e.g., loss of long-distance dispersal events); or dispersal taken over by megafauna surrogates such as livestock; or just by relying on vegetative propagation; or maybe by just being used by humans… All these situations show up eventually when one examines the natural history details of present-day “megafauna-dependent” plants. Thus, at some point it is not surprising that documented coextinctions of plants following the loss of seed dispersers are so rare, if there is any.

However, even if seed dispersal has not fully collapsed, and even if coextinctions have not been extensive, the consequences have been non-trivial for the plant species that lost their megafauna frugivores: increased clumping, increased population isolation, severily-limited gene flow via seed, loss of genetic diversity, markedly reduced effective population sizes (i.e., the number of adults effectively contributing progeny), and demographic bottlenecks. Much research is still needed to fully understand which are these “cryptic” consequences of collapsed seed dispersal mutualisms, yet there are good evidences that the demographic and population genetic consequences are non-trivial.
Collevatti, R., Grattapaglia, D. & Hay, J. (2003) Evidences for multiple maternal lineages of Caryocar brasiliense populations in the Brazilian Cerrado based on the analysis of chloroplast DNA sequences and microsatellite haplotype variation. Molecular Ecology, 12, 105–115.
Malhi, Y., Doughty, C.E., Galetti, M., Smith, F.A., Svenning, J.-C. & Terborgh, J.W. (2016) Megafauna and ecosystem function from the Pleistocene to the Anthropocene. Proceedings of the National Academy of Sciences USA, 113, 838–846.
McConkey, K.R., Brockelman, W.Y., Saralamba, C. & Nathalang, A. (2015) Effectiveness of primate seed dispersers for an “oversized” fruit, Garcinia benthamii. Ecology, 96, 2737–2747.
Hall, J.A. & Walter, G.H. (2014) Relative seed and fruit toxicity of the Australian cycads Macrozamia miquelii and Cycas ophiolitica: further evidence for a megafaunal seed dispersal syndrome in cycads, and its possible antiquity. Journal of Chemical Ecology, 40, 860–868.
Hall, J.A. & Walter, G.H. (2013) Seed dispersal of the Australian cycad Macrozamia miquelii (Zamiaceae): Are cycads megafauna-dispersed “grove forming” plants? American Journal of Botany, 100, 1127–1136.
Janzen, D.H. (1981) Enterolobium cyclocarpum seed passage rate and survival in horses, Costa Rican Pleistocene seed dispersal agents. Ecology, 62, 593–601.
Text and photos: Pedro Jordano. Seedlings and dung photos: Alicia Solana. Seed photos from Museum Goeldi Herbarium, Belém, Pará, Brazil.
Seed Dispersal Anachronisms

In 1982, Daniel H. Janzen and Paul S. Martin advanced the hypothesis that a number of plant species we see in present-day forests shows fruits and seed dispersal adaptations not consistent with their interactions with extant frugivores. Thus, only when we consider the extensive frugviory shown by the extinct Pleistocene megafauna (horses, toxodons, gomphoteres, mastodons, macrauchenias, etc.) we can understand how these species evolved the fruit traits we see nowadays. How then, did these tree and shrub species persist in the absence of the animal mutualists they required for population persistence? The core of the hypothesis expects these anachronic dispersal systems to be best explained by interactions with extinct animals, showing impaired dispersal resulting in altered seed dispersal dynamics.
Janzen and Martin defined seed dispersal anachronisms as those dispersal syndromes with fruit traits and phenological patterns best explained by interactions with extinct animals and offered some striking examples of Neotropical fruits with anachronic traits. These ‘‘unfit’’ species share fruit traits and phenological patterns that are at least in part not expected from their interactions with the extant frugivore community, but logically explained if we consider the extinction or local absence of the main frugivores.
Key traits of megafaunal fruits include 1) overbuilt design, with large seeds protected mechanically by thick and hard endocarp and indehiscence, with nutrient-rich pulp and external similarity to fruits eaten by extant large African/Asian mammals; 2) phenological segregation of ripening times throughout the year; 3) fruits falling to the ground upon ripening; 4) fruits unattractive or not very attractive to arboreal or flying frugivores; 5) a large proportion of the fruit crop rots on the tree without being consumed; 6) frugivores include a large coterie of seed predators that might act sporadically as legitimate dispersers; 7) fallen fruits are avidly eaten by introduced horses, pigs, and cattle; and 8) natural habitats of the plant species today are alluvial bottoms on gentle slopes, usually along forest edges with grassland.
It is clear that functional dispersal for many of these species operates in present-day neotropical communities by means of diplochorous and alternative seed dispersal systems involving other agents such as scatter-hoarding rodents, tapirs, cattle, some primates and even bats, as well as haphazard (runoff) and human-mediated dispersal. However, the loss of seed dispersal by extremely large mammals may imply marked shifts in the patterns and consequences of seed dispersal for these plant species. Ongoing and future research should unveil the signals of the “ghosts of past mutualisms”.
Guimarães Jr., P.R., Galetti, M. & Jordano, P. (2008) Seed dispersal anachronisms: rethinking the fruits extinct megafauna ate. PLoS ONE, 3, e1745.
Janzen, D. & Martin, P.S. (1982) Neotropical anachronisms: the fruits the gomphotheres ate. Science, 215, 19–27.
Martin, P.S. & Klein, R.G. (1984) Quaternary Extinctions: a Prehistoric Revolution. University of Arizona Press, Tucson, AZ.
How To Make A Megafauna Fruit
Just make it big, very big. Well, it’s not just that…- yet we’ll probably agree that megafauna fruits are, in some sense, overbuilt.
If we take the two major types of fruits that extant megafauna consume (e.g., elephants, rhino, etc.), we find two distinct typoplogies. Both are extremely large-sized fruits forms. The first one is like a drupaceous mega-cherry, a large fruit with a single, or very few (i.e., up to three or four) large individual seeds; the second type is kind of a mega-tomato, a large fruit with many, really many (up to hundreds), tiny seeds. Fruits of the first type are usually 4-10 cm diameter; those of the second type are usually > 10 cm in diameter.
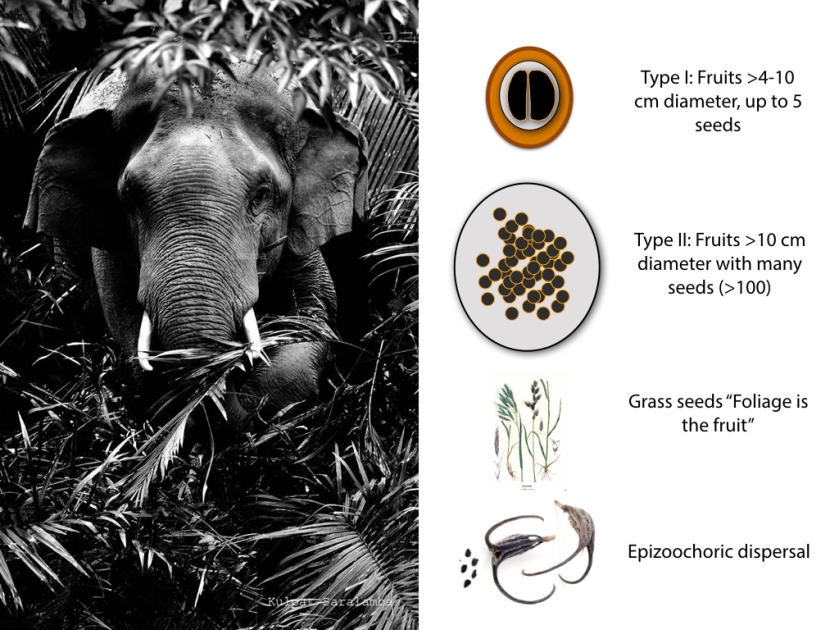
Why do these fruit types differ when compared to other ‘normal’ fuits? It is not simply that they are much larger. Their key characteristic is that, for a given number of seeds per fruit, they pack up seed sizes up to three orders of magnitude larger than ‘normal’ fruits. Thus, megafaunal fruits allowed plants to circumvent the trade-off between seed size and dispersal by relying on frugivores able to disperse enormous seed loads over long-distances.
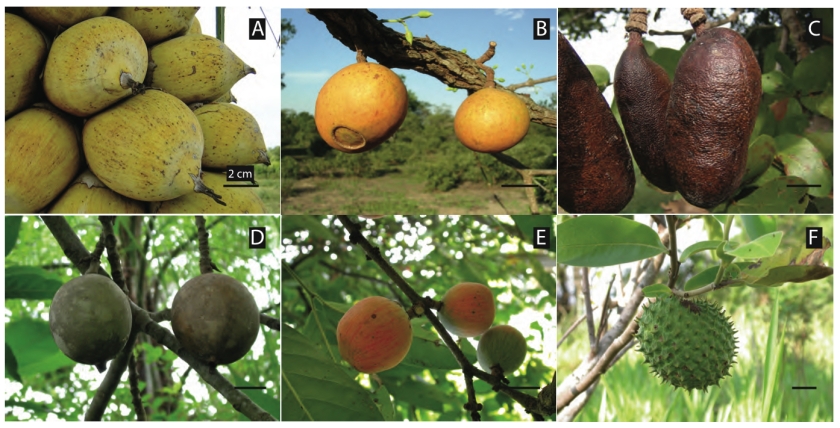
In addition to these two types of megafaunal (fleshy) fruits, the extinct Pleistocene megafauna most likely also dispersed grass seeds and seeds attached to their fur (epizoochoric).
Barlow, C. (2001) Anachronistic fruits and the ghosts who haunt them. Arnoldia, 61, 14–21.
Bretting, P.K. 1986. Changes in fruit shape in Proboscidea parviflora ssp. parviflora (Martyniaceae) with domestication. Economic Botany, 40, 170-176.
Feer, F. (1995) Morphology of fruits dispersed by African forest elephants.
African Journal of Ecology, 33, 279–284.
Guimarães Jr., P.R., Galetti, M. & Jordano, P. (2008) Seed dispersal anachronisms: rethinking the fruits extinct megafauna ate. PLoS ONE, 3, e1745.
Janzen, D. & Martin, P.S. (1982) Neotropical anachronisms: the fruits the gomphotheres ate. Science, 215, 19–27.
Janzen, D. (1984) Dispersal of small seeds by big herbivores: foliage is the fruit. American Naturalist, 123, 338–353.
Extinct Megafauna Frugivores
The diversity of extinct megafauna frugivores was extremely high in different continents, and a number of them played a central role in the evolution of fruit traits we see today. While the largest South American extant mammal is the tapir (Baird’s, up to 400 kg), consider that 100% of megamammal species (body mass >1000 kg) and about 80% of large mammal species (those over 44 kg) from the Pleistocene South American fauna was extinct ca. 10-12 Kyr BP. At least 37 genera of mammals were eliminated, including most of the megafauna species (i.e., gomphotheres, camelids, ground sloths, glyptodonts, and toxodontids). All megamammals (37 species) and most large mammals (46 species) present during the late Lujanian (latest Pleistocene- earliest Holocene) became extinct in South America (around 30 genera of mammals vanished in North America, 17 in Australia, and 24 in Asia). In contrast, Africa lost 8 of 50 megamammal genera. Africa and Southern Asia are the only continental areas that have terrestrial mammals weighing over 1000 kg today.
A number of the extinct Pleistocene megamammals were herbivores, grazers, browsers, and certainly did include fruits in their diet, probably in large amounts, thus potentially acting as seed dispersers for a variety of plants. This fact has been evidenced from coprolites and isotopic analysis of fossil remains, with additional insight from comparative anatomy and morphology. Present-day plant-frugivore interactions still have the signals of these ghosts of evolution.
Haynes, G. (ed.). 2009. American megafaunal extinctions at the end of the Pleistocene. Springer, Berlin.
Barlow, C. 2000. The ghosts of evolution: nonsensical fruit, missing partners, and other ecological anachronisms. Basic Books, New York.
Illustration: Sinammonite @deviantart.com
Most of the species shown in this great illustration from Sinammonite (http://sinammonite.deviantart.com/) were frugivorous (probably with the exception of the large carnivores) and legitimate seed dispersers of their food plants. The figure is high-res; you may wnat to zoom-in and seek the species names by the numbers.
Prehistoric megafauna.
1. Chilotherium anderssoni: 1.4m
2. Ancylotherium sp.: 1.8m
3. Sinotherium lagrelii: 2.6m
4. Pachycrocuta brevirostris: 1m
5. Panthera tigris: 0.97m
6. Homotherium crenatidens: 1.1m
7. Xenosmilus hodsonaei: 1.1m
8. “Amerhippus” scotti: 1.5m
9. Hipparion insperatum: 1.9m
10. Loxodonta atlantica: 3.5m
11. Stegodon zdanskyi: 3.9m
12. Ningxiatherium euryrhinu: 2.2m
13. Gigantopithecus blacki: 1.8m
14. Dinocrocuta gigantea: 1.4m
15. Amphimachairodus palander: 1.1m
16. Smilodon populator: 1.2m
17. Panthera atrox: 1.3m
18. Equus sussenbornensis: 1.8m
19. Elephas maximus: 2.9m
20. Dzungariotherium orgosense: 4.5m
21. Palaeoloxodon antiquus: 4m
22. Bison priscus: 2.1m
23. Equus capensis: 1.46m
24. Elasmotherium chaprovicum 2.8m
25. Mammuthus trogontherii: 4.5m
26. Proboscidipparion sinense: 1.8m
27. Plesippus enormis 1.65m
28. Ceratotherium cottoni: 1.8m
29. Diprotodon optatum: 1.9m
30. Palaeoloxodon recki: 4.5m
31. Mammut borsoni: 3.5m
32. Equus koobiforensis: 1.6m
33. Palorchestes azael: 1.3m
34. Sivapanthera pleistocaenica: 1m
35. Equus mosbachensis: 1.65m
36. Stephanorhinus kirchbergensis: 2m
37. Palaeotherium giganteum: 1.5m
38. Zygomaturus trilobus: 1.5m
39. Deinotherium giganteum: 3.5m
40. Paraceratherium lepidum: 4.5m
41. Mammuthus columbi: 4m
42. “Equus” major: 1.78m
43. Coelodonta antiquitatis: 1.8m
44. Bubalus youngi: 1.8m
45. Dzungariotherium? tienshanense: 5m
46. Stegodon ganesa: 4m
47. Sinohippus robustus 1.3m
48. Panthera spelaea: 1.2m
49. Embolotherium andrewsi: 2.8m
50. Allohippus sanmeniensis: 1.8m
51. Elasmotherium caucasicum: 3m
52. “Equus” giganteus: 2.25m
53. Syncerus antiquus: 1.65m
Seed dispersal by megafauna (extinct and extant)
I’ll be posting a series on megafauna (extinct and extant) and megafauna-dependent plants that I’ve been contributing to our Facebook page Frugivores & Seed Dispersal during the month of December. The posts focused on megafauna frugivores and megafauna-dependent fruits and seeds, and the processes of dispersal associated with them. I also included other interesting posts on frugivory and seed dispersal, as ever, but megafauna was the focus. Hopefully we contribute to a better appreciation of the distinct ecological roles and the contribution of megafauna species to the functioning and maintenance of ecosystems around the world, specifically on their role as frugivores and seed dispersers.
Among the most spectacular frugivores and seed dispersers we find the Megafauna species, those amazing beasts that impress every naturalist because of their adaptations, life histories, and specific traits. Yet megafauna species are being particularly hard hit by human-driven activities, notably hunting and deforestation. Megafauna species are traditionally defined as being above 40 kg body mass (i.e., > 100 lb), and include a full range of mammals (e.g., rhino, elephants, a number of antelopes, large primates), birds (e.g., ostrich, cassowary, emu), and reptiles (e.g., varanids, turtles). Moreover, think about the late Pleistocene (~12 Kyr BP) extinction of an even richest diversity of megafauna species: toxodons, terrestrial sloths, mamuths, gliptodons, gomphoteres, etc. The study of frugivory and seed dispersal (FSD) by megafauna opens a number of extremely interesting questions, ranging from the role of past history in shaping fruit traits, the lasting signatures of past extinctions of major seed dispersers for plants (e.g., in the genetic pools), the conflicts and interactions with humans in natural and seminatural habitats, the role of extremely long-distance seed dispersal by megafauna and its collapse following extinction, etc.
Illustration: Dadi, “Cada um”.
Chasing interactions
Ecological interactions are the wireframe of biodiversity. No single species on Earth lives without interacting with other species. Thus, biodiversity is more than just species: interactions among them are the architecture that supports ecosystems. It’s the Web of Life.
Just in the same way we sample individuals of free living species to estimate the diversity of a particular area or ecosystem, we can sample interactions. In this way we can assess the full complexity of ecosystem structure.